Exploring the Application of Non-Newtonian Fluids in Aviation
- Your Tutor TCC
- Aug 27, 2024
- 5 min read

To estimate the impact absorption capacity of a non-Newtonian liquid during a collision between a plane and the ground, several factors need to be considered, including the forces involved, the properties of the non-Newtonian liquid, and their interactions.
First, the kinetic energy of the plane at the moment of impact must be calculated.
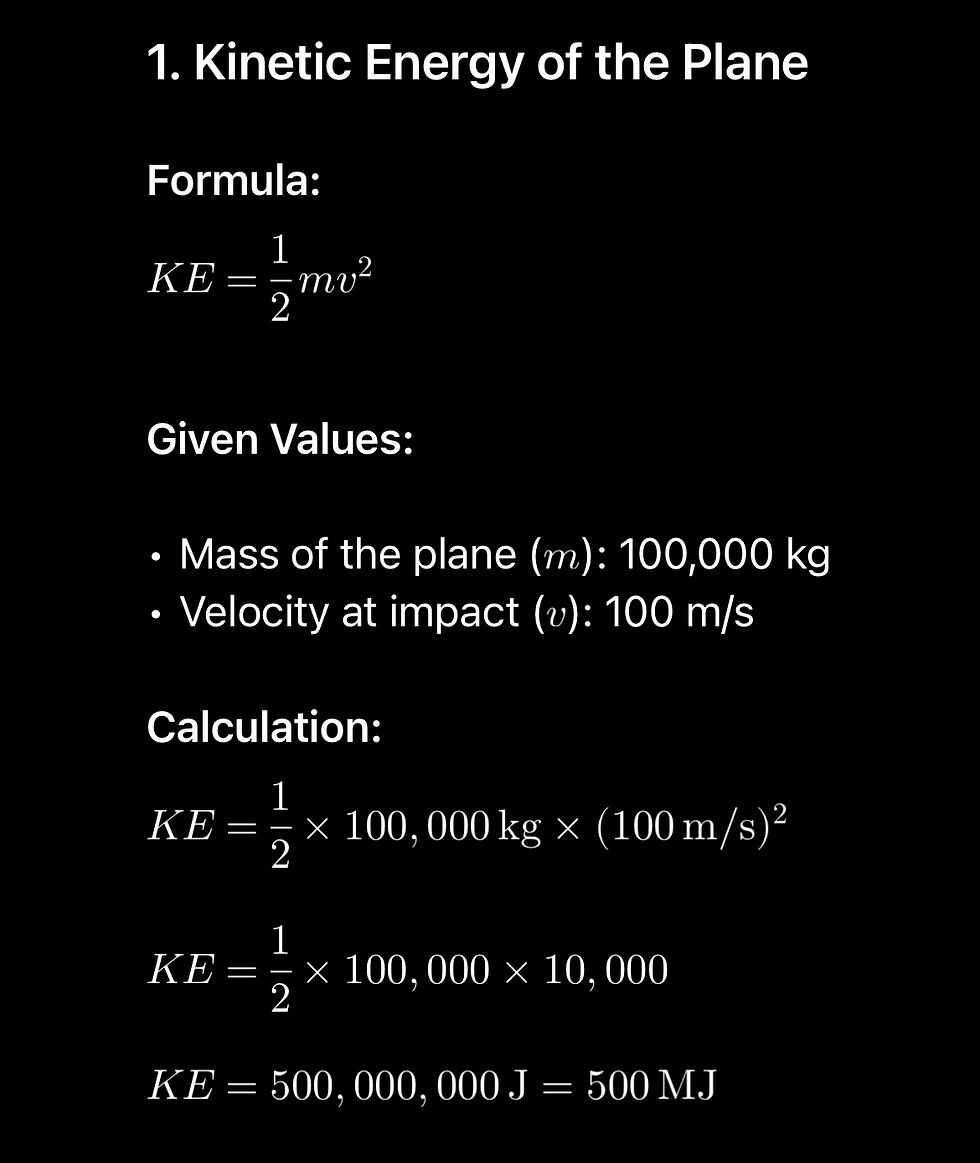
Next, the force of impact can be estimated using the work-energy principle.
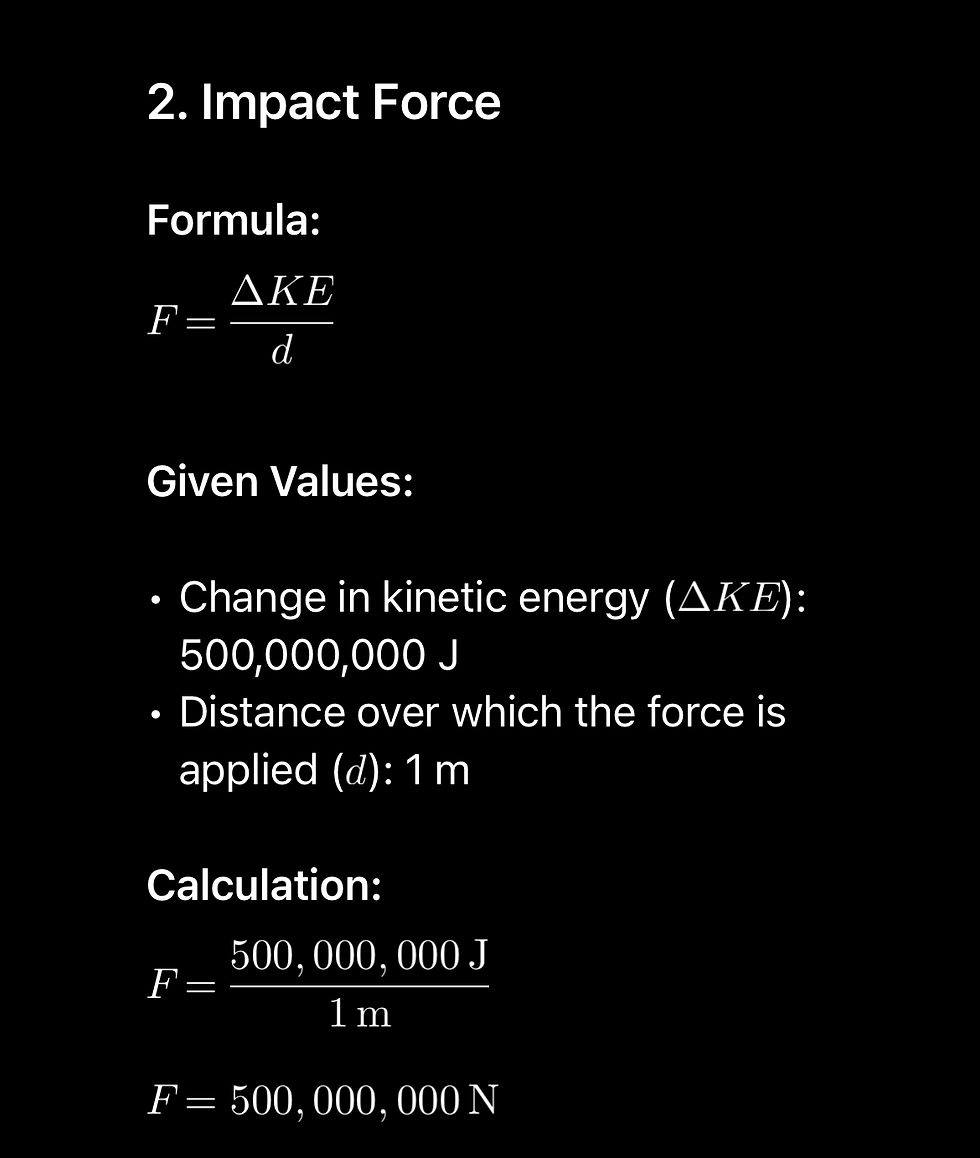
The properties of the non-Newtonian liquid are crucial in this simulation. Non-Newtonian liquids exhibit shear-thickening behavior, meaning their viscosity increases with the rate of shear stress. This allows them to absorb a substantial amount of energy during impact. To model this behavior, data on the liquid's viscosity under high shear stress is necessary, which can be obtained experimentally or from studies on similar materials.
Energy absorption by the non-Newtonian liquid can be modeled by treating it as a viscoelastic material. This material dissipates energy through both elastic deformation and viscous flow. The absorbed energy can be approximated.
Newton's Second Law (\( F = ma \)) can be applied to model the plane's deceleration upon impact. Although universal gravity may not significantly affect the collision itself, it influences the plane's descent before impact.
In the simulation process, the descent of the plane is first modeled to calculate its velocity just before impact, using gravitational acceleration. Then, using this velocity and the plane's mass, the kinetic energy and impact force are determined. Finally, the non-Newtonian liquid's response is modeled to evaluate how it absorbs the impact force, considering its shear-thickening behavior.
Practical simulations require detailed material properties of the specific non-Newtonian liquid and experimental data on its high-impact behavior. Advanced computational simulations using techniques like finite element analysis (FEA) can offer more accurate predictions by modeling both the plane's structure and the liquid's response in three dimensions.
To estimate the impact energy that a non-Newtonian liquid could absorb during a plane crash, we start with some basic assumptions and calculations.
Assuming the plane is a medium-sized commercial jet with a mass of 100,000 kg and an impact velocity of 100 m/s (approximately 360 km/h), which is typical for an emergency landing, and that the non-Newtonian liquid acts as a shear-thickening fluid with a viscosity that increases significantly under impact. This liquid is estimated to absorb up to 2 MJ per cubic meter. We also assume that the plane crushes by about 1 meter upon impact.
First, we calculate the kinetic energy of the plane at the moment of impact. Substituting the given values, we find that the plane has 500 megajoules of kinetic energy at impact.
Next, we estimate the impact force. According to the work-energy principle, where the work done by the impact force equals the change in kinetic energy, we find that the estimated impact force is 500 million Newtons.
For the non-Newtonian liquid, assuming a volume of 1 cubic meter and an energy absorption capacity of 2 MJ per cubic meter, the liquid absorbs 2 megajoules of energy. This is a small fraction of the total 500 MJ of kinetic energy.
In interpretation, the non-Newtonian liquid absorbs about 0.4% of the total impact energy. This indicates that while the liquid provides some impact absorption, a larger volume or higher energy-absorbing capacity would be necessary for a more significant reduction in impact forces.
To enhance safety, increasing the volume of the liquid or combining it with other impact-absorbing materials could be effective. For instance, using 10 cubic meters of liquid could absorb up to 20 MJ, which, while still a fraction, would be more substantial. Additionally, integrating the liquid into a composite material could improve energy absorption and distribute the impact force more effectively.
To understand how a special type of liquid, known as a non-Newtonian liquid, might help absorb impact energy during a plane crash, let's break down the scenario with some simplified calculations.
First, consider a medium-sized plane with a mass of 100,000 kg, traveling at a speed of 360 km/h (approximately 100 m/s). At this speed, the plane has 500 million joules of kinetic energy. This large amount of energy results from the plane's substantial mass and high velocity.
When the plane crashes, the sudden stop creates a tremendous force on the plane and its occupants. We estimate this impact force to be around 500 million newtons. This force is responsible for causing the plane to crumple and can potentially lead to serious injuries.
The non-Newtonian liquid we are considering behaves differently when it is subjected to impact. It becomes thicker and absorbs energy more effectively under high stress. For this scenario, we assume that one cubic meter of this liquid can absorb up to 2 million joules of energy.
Given that the crash involves 500 million joules of energy, one cubic meter of the liquid, which absorbs 2 million joules, would only manage to absorb a small fraction—less than 1%—of the total impact energy. While this absorption does provide some level of protection, it is not sufficient on its own to significantly reduce the impact from a severe crash.
To enhance safety, it would be necessary to use either a larger volume of the non-Newtonian liquid or combine it with other energy-absorbing materials. For example, using ten cubic meters of the liquid could absorb up to 20 million joules, which is a more substantial amount but still a fraction of the total energy. Additionally, integrating this liquid with other protective technologies could improve overall safety by better distributing and absorbing impact forces.
In summary, while the non-Newtonian liquid has potential benefits for crash protection, using it effectively would require either increasing its volume or combining it with other advanced materials to significantly impact crash energy absorption.
In the quest to enhance crash protection in aviation through the use of non-Newtonian liquids, we can develop a comprehensive strategy that integrates current technologies, draws inspiration from ancient practices, and envisions futuristic innovations.
To start with, **current technology** can be advanced by incorporating non-Newtonian fluids into composite materials. These materials are already valued in aviation for their lightweight and strength. By embedding non-Newtonian fluids between layers of durable composites like carbon fiber, we create a material that remains flexible under normal conditions but stiffens upon impact, thus absorbing more energy and providing superior protection. Additionally, adaptive crash structures can be developed. These are designed to alter their shape or stiffness in response to stress, enabling them to better distribute and absorb crash forces, potentially reducing injuries.
Turning to ancient view, we can adopt design principles inspired by nature. Honeycomb structures, known for their strength and energy absorption, could be combined with non-Newtonian fluids inside the plane’s fuselage. These structures would collapse in a controlled way during an impact, aided by the fluid to absorb additional energy. Similarly, ancient building techniques that used materials like straw mixed with clay or sand could inform modern methods. By mixing non-Newtonian fluids with lightweight, energy-absorbing fillers, we could enhance crash resistance in critical areas of the aircraft.
Looking to the **futuristic concepts**, we might develop smart fluids integrated with AI technology. These fluids could adjust their properties in real-time based on environmental conditions such as turbulence or impending impacts. This would enable the plane to dynamically adapt to various scenarios. Another forward-thinking idea is modular crash pods. These pods, filled with non-Newtonian fluids and other energy-absorbing materials, could detach from the main aircraft and deploy safety features like parachutes to ensure passenger safety. In the far future, concepts such as force field technology could be explored, where electromagnetic fields might create protective barriers around the plane, offering a new level of impact protection.
In summary, to significantly enhance aviation safety using non-Newtonian liquids, a multi-dimensional approach is required. Short-term improvements can be achieved by integrating these fluids into current materials and structures. Medium-term strategies involve applying nature-inspired designs and ancient techniques. Long-term innovations could include advanced smart materials, modular safety pods, and potentially even force field technology. By combining these approaches, we can push the boundaries of aircraft safety and better protect passengers in the event of a crash.
Comments